Water reuse – where have we got to?
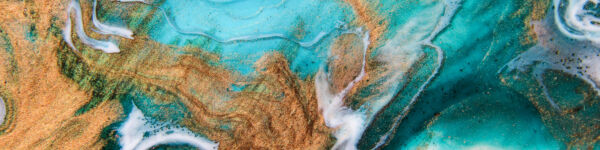
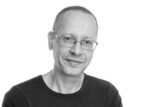
Where exactly have we got to with reusing municipal wastewater for potable water supply? How does indirect potable reuse (IPR) differ from direct potable reuse (DPR)?
Simon Judd reviews the current state of implementation, including a list of global installations.
1. What is water reuse?
Water reuse normally refers to the reclamation, purification and reuse/recycling of wastewater from municipal or industrial processes. There are three types of water reuse applications, where wastewater is recovered and reused for some purpose:
- non-potable reuse (NPR), where the recovered water is used for some purpose other than potable supply (e.g. irrigation, industrial process water, etc)
- indirect potable reuse (IPR), where water recovered from municipal wastewater passes through an ‘environmental buffer’ before being used as a supply of potable water, and
- direct potable reuse (DPR), where the recovered municipal wastewater is used for potable supply without passing through an environmental buffer.
The environmental buffer, which may be a lake, river, or a groundwater aquifer, is considered to provide additional protection through dilution or removal by filtration (for aquifers), photolysis (for surface waters), or biological degradation. Whilst this performance can be attained by additional unit process technologies for DPR, this does not address the loss in response time in the event of a process upset (such as membrane breaching or UV lamp failure). Such risks in implementing DPR must be addressed through advanced automation and real-time process performance monitoring.
2. Non-potable reuse (NPR)
NPR installations have less strict product water quality requirements than those for IPR or DPR. These schemes often employ UF or MBR followed by RO as the main purification technologies, removing both suspended and dissolved impurities. The reclaimed water is frequently used for irrigation, though other potential uses include toilet flushing and laundering (for domestic applications), fire-fighting and industrial cooling or processes.
Feedwater sources for NPR may be either industrial effluent or municipal in origin. Reclaimed industrial effluents are normally reused on site as process waters in place of mains water. Recovered municipal waters may be employed either for industrial process water, irrigation, or other municipal or community applications.
2.1 Sulaibiya
The Sulaibiya plant in Kuwait is the largest NPR plant in the world with a feed capacity of 425 MLD (megalitres/day) and a potential treated water production rate of 319 MLD. The feed municipal wastewater is transported 25 km from the preliminary treatment works at Ardiya (comprising coarse screening and degritting) to the MLE-configured activated sludge plant at Sulaibiya, before going to the UF−RO reclamation plant (Fig. 1).
The UF was originally based on capillary tube technology, employing 8,704 modules of 35 m2 each yielding a total UF membrane area of 304,640 m2 (68 skids fitted with 32 membrane housings containing four membrane elements). The downstream RO plant comprises 42 skids in a 4:2:1 array of pressure vessels (PVs) each containing seven RO modules. This yields 21,168 elements in total of 37 m2 membrane area each, hence 783,216 m2 total RO membrane area. The total project cost was $442m.
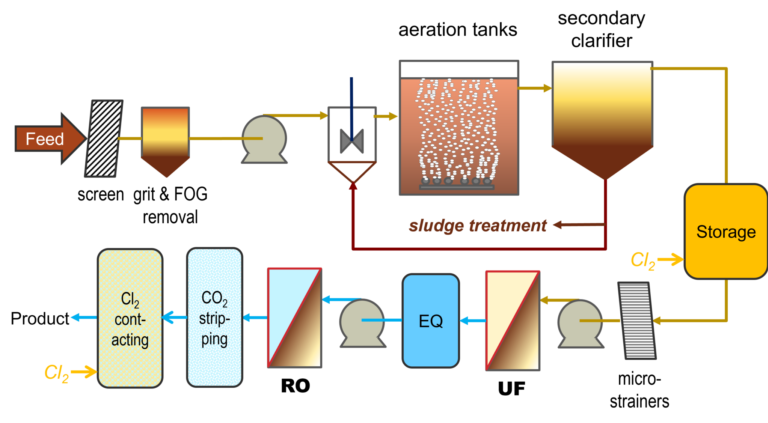
2.2 Gippsland Water Factory, Australia
The 35 MLD Gippsland Water Factory generates 8 MLD of reclaimed water from domestic (16 MLD) and pulp and paper (19 MLD) wastewater streams. The scheme (Fig. 2) incorporates a number of unusual and ambitious features, including biological treatment of both the water and off gas streams, and has been operating since 2010.
The treatment scheme includes preliminary treatment, anaerobic treatment, primary sedimentation, MBR treatment, biological nutrient removal, disinfection and RO. The scheme has segregated municipal and industrial wastewater streams, the waste activated sludge and industrial wastewater being treated in anaerobic lagoons (AL) prior to biological treatment via an MBR which then also oxidises the anaerobically-generated H2S to elemental sulphur. Segregation of the higher salinity and more highly-coloured industrial effluent stream is critical in reducing the overall load on the MBR, and also in protecting the RO downstream of the municipal MBR component. The design also permits flexible use of the MBR membranes during peak municipal flows of up to 40 MLD, when all 12 of the cells can be harnessed to treat the flow rather than the 4 assigned to average daily flow conditions.
Each of the 12 cells are fitted with 7,220 m2 of membrane area (Daigger et al., 2013), provided by 216 membrane modules, yielding a peak flux of 19 LMH at 40 MLD flow. With the normal configuration, employing eight of the cells for treating 19 MLD of industrial effluent, the industrial MBR flux is around 14 LMH. Extensive pilot trials demonstrated that anaerobic pre-treatment reduced the fouling propensity of the downstream aerobic MBR membranes.
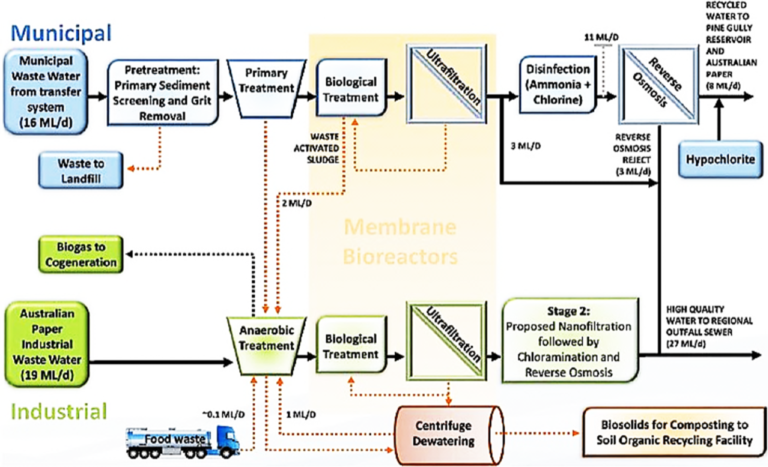
2.3 Other installations
NPR plants are also commonly installed on industrial sites, the treated water being recycled to the industrial process. Examples include the Kanes MBR−RO installation at the company’s site at Evesham in the UK and the Pasfrost UF−RO water reclamation facility in Passendale in Belgium. Both of these sites carry out vegetable processing, which incurs a high water demand associated with product washing; the motivation for reuse is to reduce the consumption of mains water.
3. Indirect and direct potable reuse (IPR and DPR)
3.1 Reuse parameters and important considerations
Key parameters in reclaiming wastewater for potable supply comprise (Fig. 3):
- the legislated maximum contaminant concentration (MCL) of chemical pollutants in the treated water, as well as the process performance in terms of pathogen removal expressed as the log removal value (LRV)
- the minimum contaminant concentration measurable (MRL, the method reporting limit)
- the frequency of events (process failure and mitigating factors, including environmental) leading to degradation of treated water quality or some other onerous outcome (such as pollution from residuals), and
- the impact of treated water quality degradation, or possible pollutant release, associated with the above events.
The risk of pathogen breakthrough for specific unit processes can be determined by quantitative microbial risk assessment (QMRA), which requires extensive data sets for both the source water pathogen concentrations and the performance of the unit process. In particular, data on the likelihood, nature and impact of process failure (or 'hazard events') on downstream pathogen levels is required.
Environmental impact assessment (EIA) becomes important when:
- the environmental buffer used is uncontained, such as a river or canal, and/or
- waste streams generated, such as reverse osmosis concentrate, are discharged to the environment.
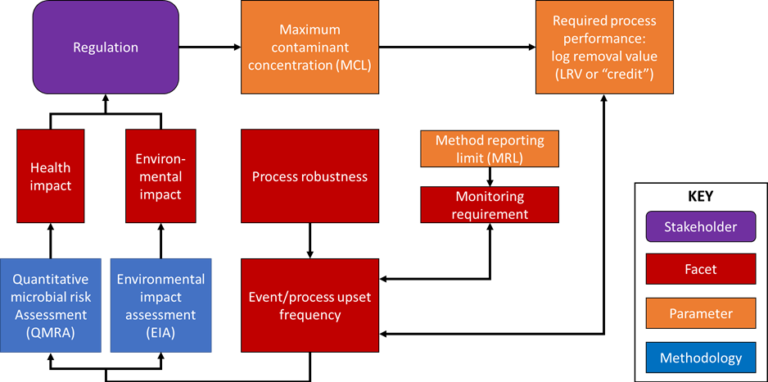
3.2 Potable reuse installations
Installations dedicated to wastewater reuse for potable supply are predominantly US-based (Table 1, in two parts below). There are additionally multiple installations established in Australia, Singapore, and Southern Africa.
The oldest potable water reuse scheme in the world is the Goreangab Water Reclamation (DPR) Plant at Windhoek, Namibia (Fig. 4). This plant is fed from an existing maturation pond and comprises nine predominantly physicochemical treatment steps – including two ozonation stages and an ultrafiltration step. The largest projected scheme is the Tuas Water Recycling Plant in Singapore, a new-build MBR-based scheme (Fig. 5) designed to recover most of the water and resources from 650 and 150 MLD of domestic and industrial wastewater streams respectively (Zheng, 2021).
Table 1 (Part 1). Drinking water reuse installations, >1 MLD capacity, IPR unless otherwise stated (Jeffrey et al, 2022)
“e” expanded; GWE Groundwater recharge; SWA/R Surface water augmentation/recharge; SAT soil-aquifer treatment; DI direct injection; SB seawater barrier; SG spreading ground; RBF riverbank filtration; C/AWT conventional/advanced water treatment; BAC biological activated carbon; Cl chlorination; DAF dissolved air flotation; GAC granular activated carbon; IX ion exchange; LC lime clarification; MBR membrane bioreactor; MF microfiltration; O3 ozone Disinfection; PAC powdered activated carbon; RO reverse osmosis; UF ultrafiltration; UV ultraviolet radiation; CSF continuous sand filtration; BW desalinated brackish water
1Superceded by GWRS; 2demonstration project: not put into service 3Intermittent operation on demand; 4Untreated wastewater used primarily for agricultural irrigation
Table 1 (Part 1). Drinking water reuse installations, >1 MLD capacity, IPR unless otherwise stated (Jeffrey et al, 2022)
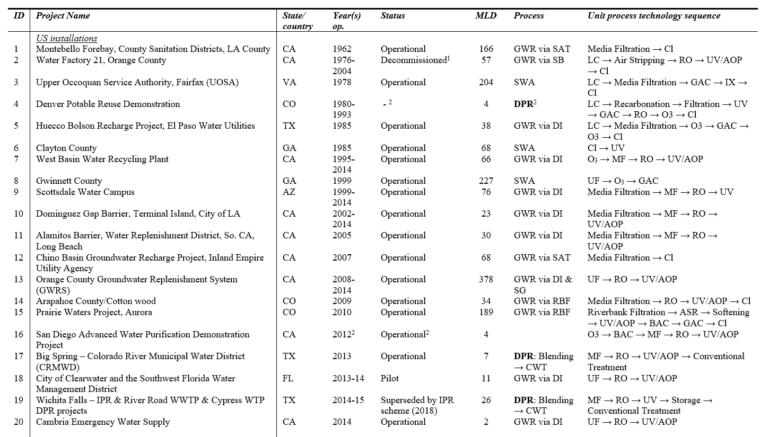
“e” expanded; GWE Groundwater recharge; SWA/R Surface water augmentation/recharge; SAT soil-aquifer treatment; DI direct injection; SB seawater barrier; SG spreading ground; RBF riverbank filtration; C/AWT conventional/advanced water treatment; BAC biological activated carbon; Cl chlorination; DAF dissolved air flotation; GAC granular activated carbon; IX ion exchange; LC lime clarification; MBR membrane bioreactor; MF microfiltration; O3 ozone Disinfection; PAC powdered activated carbon; RO reverse osmosis; UF ultrafiltration; UV ultraviolet radiation; CSF continuous sand filtration; BW desalinated brackish water
1Superceded by GWRS; 2demonstration project: not put into service 3Intermittent operation on demand; 4Untreated wastewater used primarily for agricultural irrigation
Table 1 (Part 2). Drinking water reuse installations, >1 MLD capacity, IPR unless otherwise stated (Jeffrey et al, 2022)
“e” expanded; GWE Groundwater recharge; SWA/R Surface water augmentation/recharge; SAT soil-aquifer treatment; DI direct injection; SB seawater barrier; SG spreading ground; RBF riverbank filtration; C/AWT conventional/advanced water treatment; BAC biological activated carbon; Cl chlorination; DAF dissolved air flotation; GAC granular activated carbon; IX ion exchange; LC lime clarification; MBR membrane bioreactor; MF microfiltration; O3 ozone Disinfection; PAC powdered activated carbon; RO reverse osmosis; UF ultrafiltration; UV ultraviolet radiation; CSF continuous sand filtration; BW desalinated brackish water
1Superceded by GWRS; 2demonstration project: not put into service 3Intermittent operation on demand; 4Untreated wastewater used primarily for agricultural irrigation
Table 1 (Part 2). Drinking water reuse installations, >1 MLD capacity, IPR unless otherwise stated (Jeffrey et al, 2022)
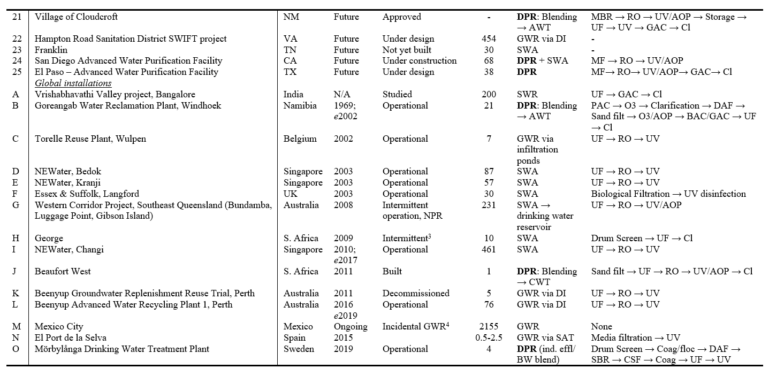
“e” expanded; GWE Groundwater recharge; SWA/R Surface water augmentation/recharge; SAT soil-aquifer treatment; DI direct injection; SB seawater barrier; SG spreading ground; RBF riverbank filtration; C/AWT conventional/advanced water treatment; BAC biological activated carbon; Cl chlorination; DAF dissolved air flotation; GAC granular activated carbon; IX ion exchange; LC lime clarification; MBR membrane bioreactor; MF microfiltration; O3 ozone Disinfection; PAC powdered activated carbon; RO reverse osmosis; UF ultrafiltration; UV ultraviolet radiation; CSF continuous sand filtration; BW desalinated brackish water
1Superceded by GWRS; 2demonstration project: not put into service 3Intermittent operation on demand; 4Untreated wastewater used primarily for agricultural irrigation
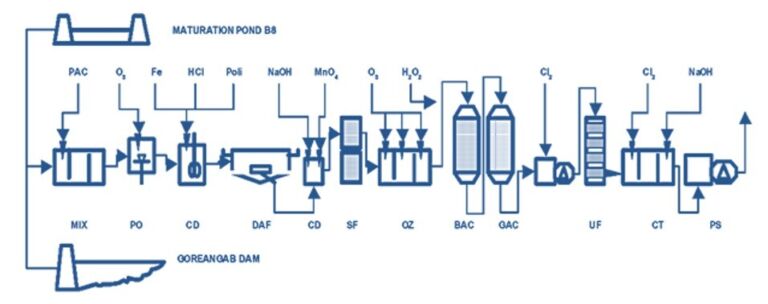
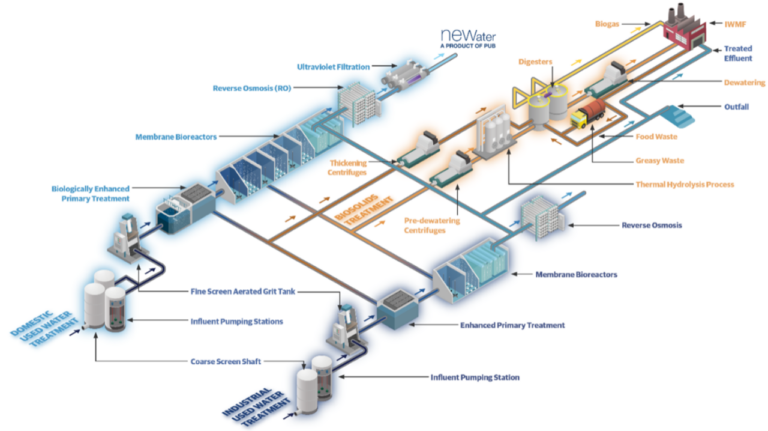
There are a number of common features across the IPR schemes summarised in Table 1:
- The environmental buffer in IPR schemes is most often groundwater (groundwater recharge, GWR), which generally allows extended times for attenuation of residual pollutant levels. Surface water augmentation (SWA) is also employed, but normally as a consequence of the GWR option being unavailable.
- The use of RO followed by UV irradiation, either for disinfection or occasionally as part of an AOP, is widespread: more than half the IPR/DPR schemes employ this combination of unit operations. RO requires protection from channel blockage by fine solids and colloids, most often achieved by UF or, more recently, MBR technology.
- The few DPR schemes employ supplementary treatment steps and/or incorporate engineered storage to provide attenuation.
4. Synopsis and outlook
There exists a key distinction between water reuse applications. For non-potable reuse (NPR) of the reclaimed water – for such duties as irrigation, industrial process water, cooling or boiler feed water – process failure impacts are largely economic in nature with minimal or no human health implications. Potable water reuse, where municipal wastewater is recovered for potable water supply, demands extremely high product water quality with commensurately low risk of lapses in quality due to potential impacts on human health.
NPR is driven by economic considerations or the conservation of freshwater supplies (which may in itself be determined by economics). There are increasing numbers of industrial effluent reuse schemes where the recovered water is reused in either on-site utilities or for the industrial process itself (i.e. recycling).
There is continued implementation of indirect and direct potable reuse schemes (IPR and DPR), which are of higher public profile. Heath and safety concerns over such schemes appear unjustified. Exhaustive studies of possible negative health impacts of recovered water for drinking have revealed these to be negligible and/or comparable with a conventional potable water supply. Such investigations have included:
- early epidemiological studies of possible pathogen-related acute conditions, and diarrhoeal disease specifically
- recent vitro bioassays aimed to account for possible chronic conditions associated with very low levels of potentially bio-active micropollutants, often termed ‘chemicals of emerging concern’ (CECs)
- direct assay of water for CECs, including perfluorinated ‘forever’ chemicals, recognised as having long-term onerous human health and/or environmental impacts, and
- comparison of the health risk incurred by de facto (i.e. unintentional) reuse of municipal wastewater for potable water supply – which has been shown to present a substantially greater risk to health than either IPR or DPR.
Given the increasing stresses on freshwater supply it is inevitable that implementation water reuse schemes will continue apace, including for potable water supply. The long-recognised challenge of engaging the public appears to be greater than the technical challenge of ensuring a reliably high product potable water quality. Future schemes may also aim to recover other resources from the wastewater, as is the case for the planned installation at Tuas, to reduce the environmental footprint.