Chasing alternative solutions in wastewater treatment
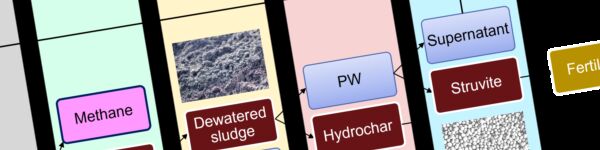
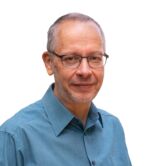
Simon Judd examines the advances made in wastewater treatment and resource recovery over the past few years with reference to five selected technologies. It is concluded that widespread implementation of many of these alternative technologies developed is ultimately constrained by cost and practicalities, though ensuring sustainability remains imperative.
1. Why develop new wastewater technologies?
There are a multitude of novel wastewater treatment technologies or processes in the municipal sector which have been investigated and developed over the past 5−10 years. These are, on the whole, intended to supplement, improve or displace conventional aerobic treatment to reduce energy consumption and/or permit resource recovery, thereby ensuring a more sustainable process.
It is a matter for debate as to how many of them could be described as truly ‘innovative’: to qualify as such they have to have been implemented and made a significant societal change. There are nonetheless some novel wastewater and sludge treatment concepts that have been developed to the point where they have been at least successfully demonstrated at pilot or full scale, if not implemented. Below are just five of these, randomly selected and listed in no particular order, all of which reduce the overal energy consumption and/or achive a degree of resource recovery, and have reached varying levels of implementation. The key features and constraints of each are briefly identified.
2. Example technologies
2.1 Anaerobic wastewater treatment
Anaerobic digestion of sewage sludge has been widely implemented for 30 years or more. The process converts the organic carbon to methane, as opposed to the carbon dioxide product generated by aerobic treatment. AD also stabilises the sludge, reducing the odour and putrefaction, and decreases the volume generated. The relatively high levels of organic matter in sludge mean that significant quantities of methane are produced, which can be captured and used to generate energy.
The viability of anaerobic processes for treating wastewater, rather than sludge, depends on the organic carbon load and the ambient temperatures. The higher the organic carbon concentration, the greater the production of methane. The added value provided by the methane then offsets the higher capital costs of the process compared with aerobic treatment, associated with the longer residence times, the required covering of the tank and the management/purification of the offgas. Many industrial effluents have high associated organic carbon loads, such that anaerobic MBRs (anMBRs) have been successfully implemented for such wastewaters. Anaerobic processes do not nitrify, and so AnMBRs are most suited to effluents with low ammonia concentrations, such as from food and beverage production.
Anaerobic treatment of municipal wastewater has been extensively implemented in warm climates for municipal wastewater treatment: there are hundreds of UASB (upflow anaerobic sludge blanket clarifier) installations in Brazil alone. These require downstream aerobic treatment, often using trickling filters (Fig. 1), to remove residual organic carbon and ammonia. Whilst UASBs provide the capability of recovering methane, the quantities produced are generally small and the offgas is often flared without being used.
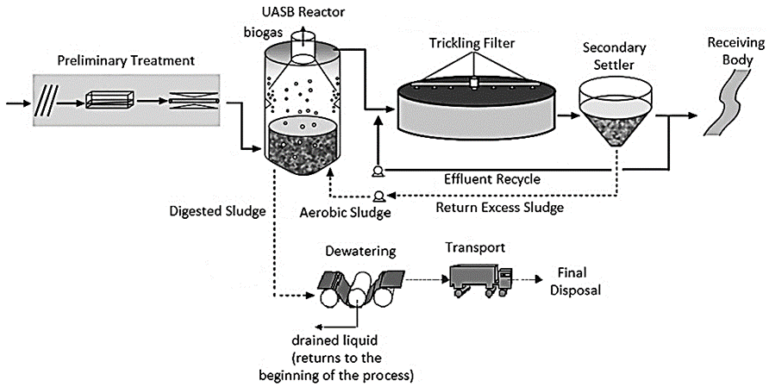
As well as the high capital costs, there is also the challenge imposed by the solubility of methane in water. Although this is low, it increases with decreasing temperature. Methane is a significant greenhouse gas (GHG) and cannot be allowed to persist in the discharged treated effluent. Thus for waters containing relatively low levels of organic carbon, specifically sewage, a greater proportion of the methane generated is dissolved. Since the flows are also generally larger than industrial effluent flows, the total amount of dissolved methane can be significant and must be stripped from the water and recovered for energy generation. This stripping further adds to the process costs. This requirement, along with the lower temperatures and negative impacts of sewage dilution, significantly challenge the potential cost benefit of implementing anaerobic sewage treatment in colder climates.
2.2 Phosphate recovery
Phosphorus (P) is most commonly removed from municipal wastewater by chemical dosing with ferric or alum coagulants, i.e. by chemical phosphate removal (CPR), forming phosphate salts. It can subsequently be extracted from sewage sludge, or any subsequent solid waste stream where it is highly concentrated (including anaerobic digestate and incinerator ash), relatively simply by acid leaching.
P can also be recovered from liquid concentrates generated from sludge processing. These generally comprise supernatants both from thickening and dewatering of the primary and waste activated sludge (WAS) streams. It can also be recovered and subsequently enriched, through membrane or adsorptive projects, from the treated sewage (Fig. 2).
There are a number of commercial processes dedicated to P recovery, ostensibly as struvite. These include:
- The Ostara Pearl® and WASSTRIP® processes offered by Evoqua (USA)
- DHV’s CrystallactorTM technology (Netherlands)
- The PhosphogreenTM and StruviaTM processes of Suez and Veolia respectively (France)
- The ANPHOS® process (Netherlands)
- Centrisys/CNP’s MagPrexTM technology (USA).
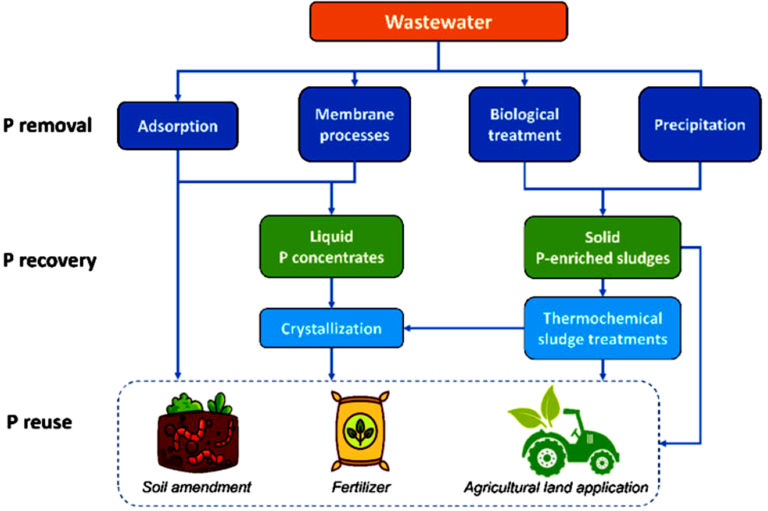
Equilibrium thermodynamics dictates that recovery is most effective from streams where P is present at the highest concentrations. This will generally be from the acid-leached sludge streams. The efficacy of the P recovery process will then depend upon whether:
- the chemical leaching, if used, can be achieved economically,
- the phosphate in the leachate or other enriched liquid streams can be both resolved from the impurities present and recovered with relatively high efficiencies,
- the subsequent recovered phosphate can be readily reused, most obviously as a fertilizer, to provide palpable value.
2.3 Hydrothermal carbonisation
Hydrothermal carbonisation (HTC) is the thermal treatment of sludge, at temperatures generally between 180 and 260°C and pressures of 10−80 bar, in the presence of water (<85%). It generates two main product streams: a solid 'biochar' (or 'hydrochar') and a liquid (often called 'process water', or PW), with a gas stream of <5% of the feed by weight. The biochar product can potentially be used either as a fuel or for soil amendment, depending on its characteristics (which in turn depends upon the sludge quality and HTC operating conditions).
There are a number of commercial suppliers of HTC technology, and a few confirmed full-scale installations. Technology providers include: Antaco (Fig. 3), HTCycle, SunCoal and TerraNova, all from Germany, along with C-Green from Finland and Ingelia from Spain. Of these providers, TerraNova appears to have an established installation, in Jining, for treating sewage sludge. The process generates a 65% dry solids product and a COD-rich PW which can be returned to the anaerobic digestor (Bajón Fernández et al, 2023).
HTC appears to present a realistic alternative to the more widely implemented incineration for resource recovery from sludge. A key advantage of the process is that there is no requirement to completely dry the feed sludge, as is the case for pyrolysis (which also generates a biochar product) or gasification (which predominantly generates a gas product). However, the PW generated then requires further treatment − though it also offers the opportunity of recovering the phosphorus and ammonia concentrated in this stream.
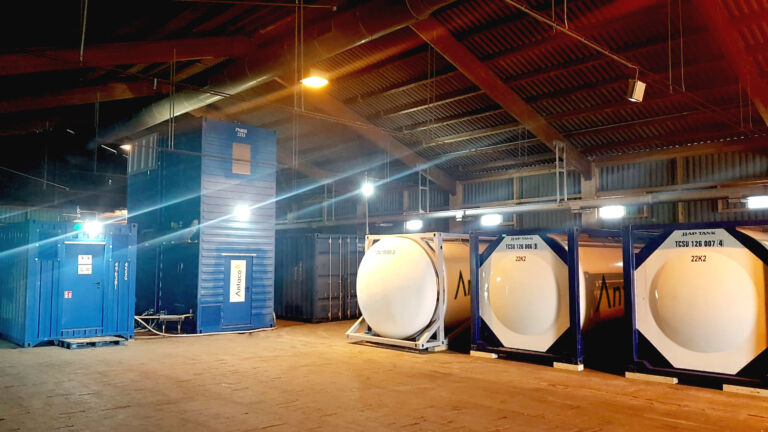
2.4 Anammox process
The potential of the anammox (the abbreviation for anaerobic ammonia oxidation) process was realised in the late 1990s when anammox bacteria were first discovered in anaerobic sludge. These bacteria are capable of directly converting ammonia and nitrite into nitrogen gas (Fig. 4), instead of requiring the conventional two-stage process of aerobic nitrification (ammonia to nitrate) and anaerobic denitrification (nitrate to nitrogen). This offers three clear advantages over the conventional nitrification/denitrification route:
- the need for an organic carbon source for nitrification is eliminated,
- the energy consumption for aeration is reduced, along with CO2 generation, and
- the excess sludge production is reduced.
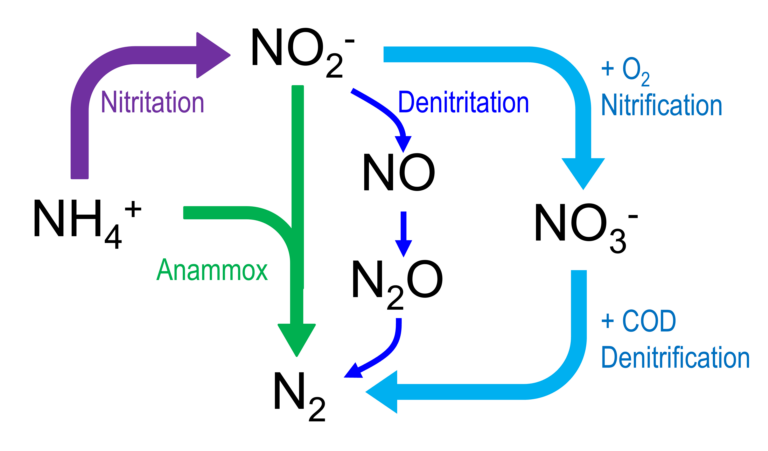
Although the advantages are clear, and a commercial system was implemented around 15 years ago by the Dutch company Paques, the process suffers from long start-up durations and sensitivity to a number of process parameters including oxygen concentration, temperature, load and wastewater chemistry. It appears to have been implemented for high-load wastewaters, and in particular landfill leachate, on a limited scale. However, the strict operation and maintenance regime, requiring rigorous control, presents a major challenge to widespread implementation.
2.5 Algal photobioreactors
The benefits of applying algae to wastewater treatment have been recognised for many years and have been widely discussed amongst the research community. Algae provide a low-energy means of removing nutrients from the wastewater. There is no requirement for aeration: algae are photosynthetic and thus need only carbon dioxide and light to sustain their growth. The resulting biomass can be processed, usually by hydrothermal liquefaction (HTL), to generate a useful bio-oil product. Algal ponds or photobioreactors (PBRs, Fig. 5) thus represent a potentially energy-neutral option for wastewater treatment.
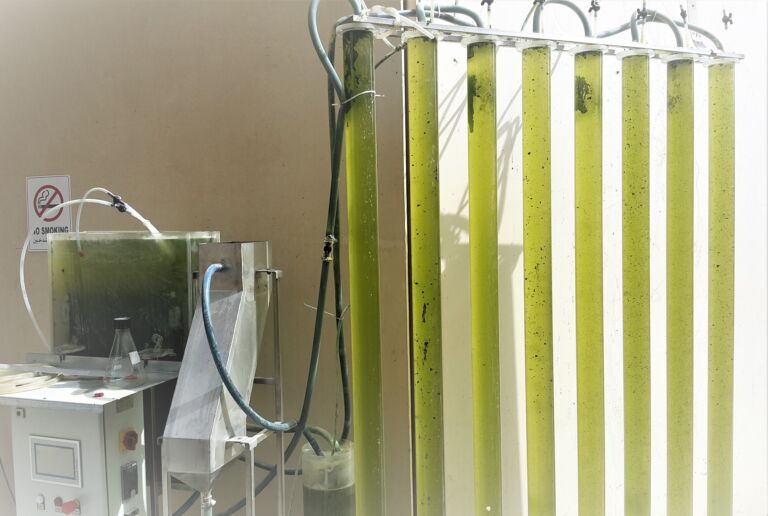
There are, however, significant impediments to the widespread implementation of algal PBRs for wastewater treatment specifically. These are primarily associated with:
- the need for daylight as the energy source for photosynthesis, which has to penetrate the biomass, and
- the slow growth of the algal cells.
The demand for light as a power source means that the biomass depth has to be sufficiently shallow (preferably 10−20 cm) to allow reasonable light penetration. This, combined with the low growth rate – around 5−10 times lower than for a classical aerobic process – means that the footprint of a standard 'high-rate' algal pond for wastewater treatment is correspondingly at least an order of magnitude greater.
The alternative to a pond-based system is to use an array of tubes through which the algal biomass can be passed. However, this then incurs a significant capital cost associated with the tubes.
Further costs are incurred by the recovery of the bio-oil from the algal biomass. The cost benefit of this step is strongly associated with the bulk cost of mineral oil.
2.6 Other technologies
There are a great many other wastewater treatment technologies or processes which have received considerable attention by the research community. These include microbial fuel cells, biological hydrogen production and osmotic membrane bioreactors.
In the first two cases, and others where renewable energy is being targeted, the economic viability of the process increases with increasing organic carbon load. Thus, as with anaerobic treatment of wastewater, the technologies are more suited to industrial effluents from, say, the food and beverage industries than for municipal wastewater.
OMBRs (Fig. 6) employ novel forward osmosis membranes that have already been commercialised. However, it is debatable that this hybrid of forward osmosis (FO) and MBR technology offers a clear advantage over the conventional two-stage MBR–reverse osmosis (RO) process that has been implemented for water reuse. There also appears to have been very limited implementation of FO technology per se, mainly due to the challenge of recovering the draw solution.
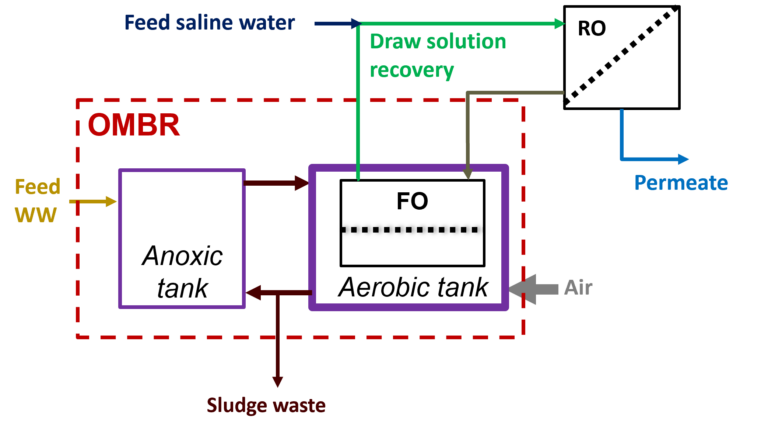
A large number of other novel membrane materials have been developed, perhaps most notably graphene/graphene oxide membranes and carbon nanotubes. These materials offer extremely high water permeabilities. However, despite the wealth of research conducted and the existence of a few start-ups, there appear to be no examples of implementation of these membranes which are challenged by difficulties with:
- achieving uniformity in the quality of supply of the bulk raw material (such as graphene oxide),
- continuous fabrication of large membrane areas (>1 m2), and
- establishing the long-term stability of the formed membranes, especially with respect to chemical cleaning.
3. Summary
It is undeniably the case that there have been some very elegant technological solutions developed to tackle the world’s burgeoning crisis of unsustainability. These can be combined to produce a treatment scheme where a number of different resources are recovered (Fig. 7).
However, many of the technologies have, at their root, their own sustainability challenge. These challenges are manifested as an economic and/or environmental penalty – where implementation of the technology exerts its own negative environmental impact. Having said this, there is no option but to continue to explore these alternative solutions. Many existing wastewater and waste management practices are unsustainable in the long term.
What seems to be needed is the identification of the pinch point of these novel approaches at an early juncture in their development. This would save time – the one thing for which there are unarguably diminishing reserves.
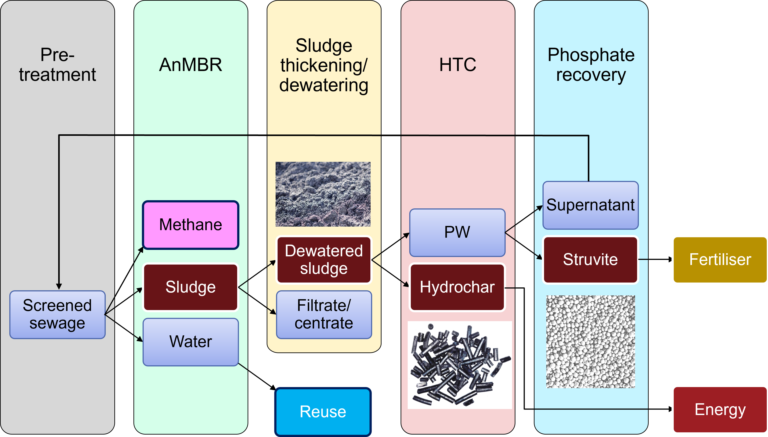