Membrane-aerated biofilm reactors (MABRs) and P removal
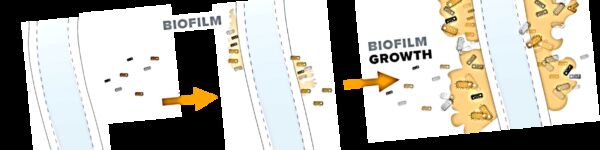
Simon Judd has over 35 years’ post-doctorate experience in all aspects of water and wastewater treatment technology, both in academic and industrial R&D. He has (co-)authored six book titles and over 200 peer-reviewed publications in water and wastewater treatment.
Barry Heffernan hosted an interesting webinar about MABRs on 8 May 2025, specifically on DuPont’s OxyMem technology. A number of points were made, but perhaps one of the most enlightening was around the impact on P removal.
1. MABRs and nitrification
Membrane-aerated biofilm reactors (MABRs) have been around for a while now. The original research into the process dates back to the early 1990s, and the first commercial technology appeared more than a decade ago. So, it hardly qualifies as a new process.
The benefits offered by the technology are well known. Chief amongst these is enhanced nitrification. Oxygen diffuses through the membrane into the biofilm, where nitrifying bacteria (Nitrosomonas, Nitrospira, etc.) oxidize ammonia (NH4⁺) to nitrite (NO2⁻), and subsequently to nitrate (NO3⁻) – all within the confines of the attached biofilm. By placing the MABR membranes in the anoxic zone of a conventional anoxic–aerobic (or oxic) biological stage, the process nitrification capacity is increased. This has been demonstrated in a number of pilot studies, as well as at full scale (as Barry was able to illustrate with data from the UK sites Spernal and Monkmoor).
But one of the most intriguing topics is the implementation of MABRs for addressing the challenges imposed by phosphorus removal.
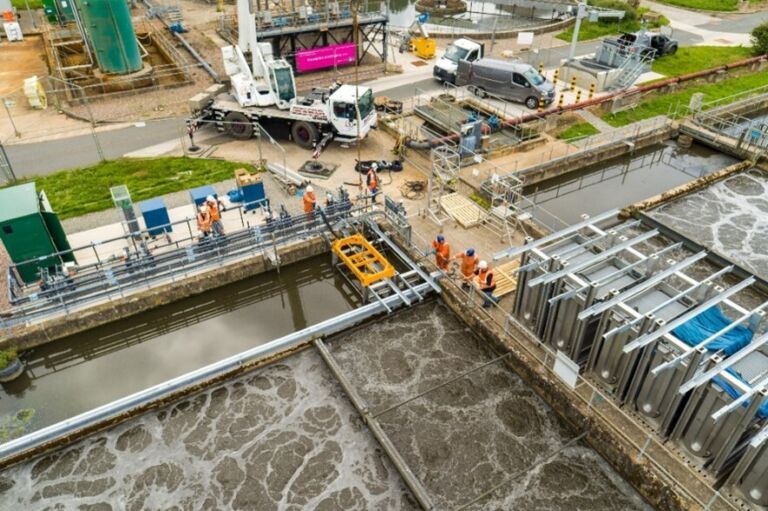
2. Phosphorus removal: chemical vs biochemical
There are basically two ways of removing phosphorus. The simple way is chemically (‘chemical phosphorus removal’ or CPR). This involves dosing with coagulant – most often ferric and usually into the aeration tank (‘simultaneous precipitation’) rather than before or after the secondary clarifier (‘post precipitation’). Simultaneous precipitation is cost-effective with reference to CAPEX and reasonably effective in terms of P removal.
The second method is to use microbiology (enhanced biological phosphorus removal or EBPR). EBPR offers a more sustainable method for removing P. The process itself demands no chemicals. Sludge production and energy consumption are both lower than for CPR. Crucially, avoiding coagulant chemicals offers the opportunity to recover P in a bio-available form, allowing it to be used as a fertiliser (struvite, apatite, vivianite, whatever). Good news for anyone selling pyrolytic processes, since the biochar generated can be used directly as a fertiliser.
3. P removal: the challenges
Whilst both CPR and EBPR both work – they unquestionably remove P – both suffer from different practical limitations.
The CPR challenge: washout
In the case of CPR, additional inorganic solids are generated from ferric dosing and precipitation of phosphorus which reduces the sludge age. Essentially, CPR demands higher waste activated sludge (WAS) rates to maintain mixed liquor suspended solids (MLSS) levels. This then causes a loss of nitrifiers (or 'washout'), effectively reducing the nitrifier solids retention time (SRT).
The EBPR challenge: footprint
EBPR relies on implementing an anaerobic step upstream of the anoxic step. This is essential since it is only under anaerobic conditions that the conversion of the wastewater volatile fatty acids (VFAs) to polyhydroxyalkanoates (PHAs) can take place. The PHAs are needed for carbon and energy storage to promote the uptake of P in the subsequent oxic step. The P is stored as intracellular polyphosphate which then ends up in the sludge. So, just by slotting in an anaerobic step, a chunk of phosphate can be transferred from the liquid to the sludge solids in fertiliser-ready form.
A key issue with retrofitting EBPR is simply one of space. The anaerobic tank has to go somewhere and, unless there’s space and funding to build a separate tank, the required space has to be purloined from the aerobic tank. This once again reduces the aerobic capacity and the associated nitrifier SRT.
4. MABRs and P removal
So, both CPR and EBPR result in a loss of nitrification – unless, in the case of the latter, a new purpose-built tank can be fitted. How can MABRs help?
Quite simply, MABRs effectively retain the nitrifiers within the system, countering the reduction of nitrifier SRT for both the CPR and EBPR processes – assuming the latter is implemented by filching the required anaerobic process volume from the aerobic tank. Restoring this SRT is critical for meeting the ammonia consent – particularly in the winter when microbiological growth is slower. According to the data from the CPR process introduced at the Monkmoor (UK) site, implementing the MABR increased the mean nitrifier SRT from 8.2 to 10.8 days – safely away from the washout SRT of ~6 days.
Of course, any other option that retains nitrifiers would be expected to have a similar effect. This includes adding media (such as for an IFAS) and using a membrane to retain the micro-organisms (such as in an MBR). But there’s something to be said for just being able to drop in what amounts to a super-efficient aerator which manipulates the biochemistry to get the job done.