MBRs and sludge membrane thickening: how thick is too thick?
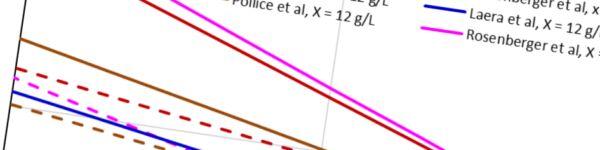
Simon Judd, Director, Judd Water & Wastewater Consultants
1. MBR operation
The various arguments surrounding the precise target sludge (or mixed liquor suspended solids, MLSS) concentration to use when running an MBR are pretty well developed, that is:
- at high MLSS concentrations (say, above 15 g/L): the risk of channel clogging is increased (which is bad), and the oxygen transfer efficiency is reduced, which increases the biological aeration energy demand (which is also bad). Against this, a higher solids concentration implies a smaller sludge volume (which is good).
- at low MLSS concentrations (say, below 8 g/L): the concentration of the foulants (extracellular polymeric species) relative to the solids is generally slightly higher, increasing the fouling propensity (which is bad), and the sludge volumes generated are higher (also bad). On the other hand, a reduced solids level reduces the process aeration energy demand because the oxygen transfer efficiency is higher (which is good).
So, as is so often the case, it’s horses for courses: the design and operating criteria selected will depend on the prevailing conditions and the specific scenario concerned.
For example, for a large works with on-site sludge processing (such as thickening followed by digestion, or dewatering followed by thermal drying or thermochemical treatment) the volume of sludge generated is likely to be of much less importance than maintaining a low energy consumption. These circumstances would then favour operation at low MLSS concentrations to reduce the specific energy consumption (the SEC per m3 of sludge) as much as possible.
For a small works, perhaps at the end of a fifteen-mile single-track road and staffed only on a Friday morning, the notion of having to frequently haul sludge to some centralised location is likely to be more onerous than a slightly increased energy consumption. Operation at a higher MLSS may increase the SEC, but if the sludge volumes are relatively small this may not be such an issue. If such operation reduces the sludge volume and the associated haulage costs then it’s likely to provide an overall cost saving.The target MLSS concentration is also impacted by the membrane and process configuration. In general, immersed hollow fibre (iHF) MBRs operate at a relatively low MLSS concentration of 8−9 g/L in the process biological tank. Immersed flat sheet (iFS) and sidestream multitube (sMT) MBRs tend to operate at slightly higher concentrations (10−11 g/L in the process tank).
For both immersed configurations, the recirculation of the sludge between the biological and membrane tanks has to be high enough to avoid a significant build-up of solids in the membrane tank. Normally the recirculation rate is 4−5 times higher than the permeation rate, and it’s this that sets the maximum MLSS concentration in the membrane tank (at 10−12 g/L in the membrane tank for an iHF and 12−15 g/L for an iFS). A simple mass balance indicates that for a recirculation rate of 4−5 times the permeation rate, the concentration in the membrane tank would be approximately 25−20% higher than the process biological tank.
So far, so what? This is all pretty much common knowledge. But it is interesting to consider what it is that ultimately sets the solids concentration range.
At the low end, there’s no major issue in operating at concentrations below 8, or even below 6 g/L, if the slightly increased fouling propensity is addressed through appropriate chemical cleaning. However, at these very low levels there’s no substantial advantage in relation to plant footprint over a conventional activated sludge process which can operate at MLSS concentrations up to 4 g/L.
So, what about the high end, when the solids concentrations approach levels associated with sludge thickening operations? There have actually been surprisingly few academic studies of the impact of really high (say >20 g/L) MLSS concentrations on operation. When MBRs were first implemented, these sorts of concentrations were regularly exceeded. Since then, with ever larger plants, the emphasis on improved energy efficiencies has led to much more conservative solids concentrations.
Anecdotal evidence from the field, plus reported pilot-scale studies (Zsirai et al, 2014), suggest that things start to go wrong at around 18 g/L: the clogging propensity increases sharply and the permeability goes down as a result (Fig. 1). Moreover, it appears that a declogged membrane doesn’t perform quite as well as one which has never clogged (Figs. 1−2), with the residual permeability following declogging and chemical cleaning decreasing with increasing extent of clogging (Fig. 2).
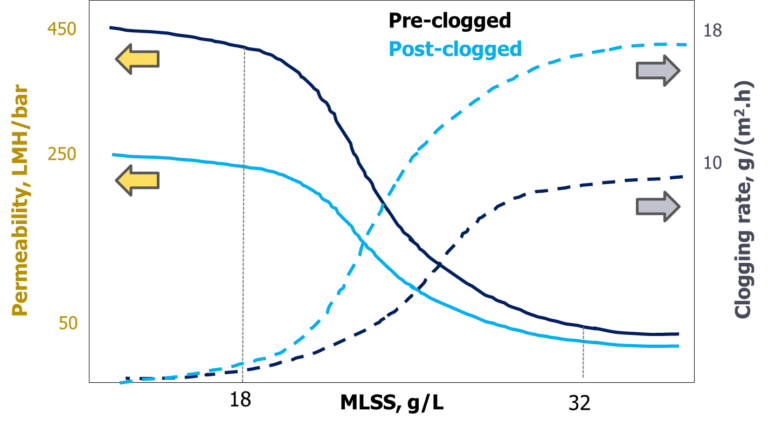
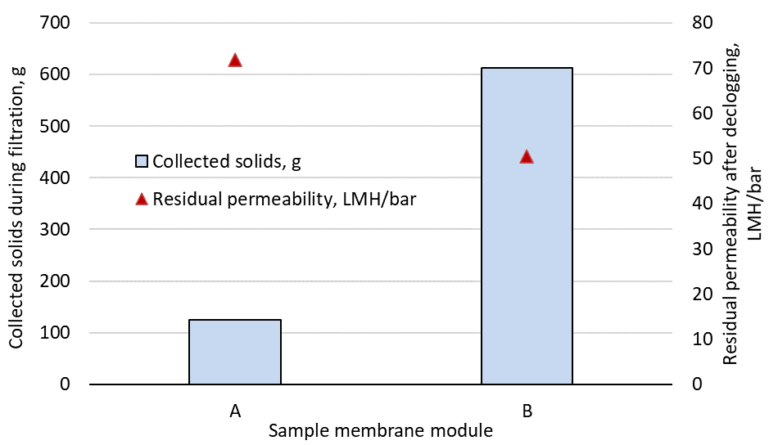
2. Membrane thickeners
There have been surprisingly few academic studies dedicated to membrane thickening of activated sludge. From work reported in this area on small-scale membrane thickening trials using immersed membranes (Wu et al, 2009; Zhu et al, 2017), it is unclear that the process has ever demonstrated to operate sustainably over an extended period. The limited evidence on this topic from the scientific literature from both these thickening studies and the MBR pilot trials conducted at high MLSS concentrations (Zsirai et al, 2014) therefore seems somewhat discouraging.
On the other hand, it is definitely possible to filter sludge solids at concentrations of 30 g/L or more. This is proven by the significant number of membrane thickeners successfully implemented alongside the membrane separation component of iFS MBRs (Woo, 2014); these thickeners use exactly the same technology as that treating the wastewater itself. The most recent membrane sludge thickeners have been based on iFS ceramic membranes (Woo, 2018), and results seem to be rather encouraging.
So, how come? How is it possible for membrane thickeners to work treating something which is apparently beyond the operating threshold of the process?
It appears that sustainable operation of membrane thickeners is critically dependent on the operating conditions. iFS membrane thickeners which have been practically implemented operate at a much lower flux (9−12 LMH) and a higher air scour rate than iFS MBRs. This perhaps just comes down to common sense. If the solids concentration has gone up, the flux has to be reduced to maintain the transport of solids to the membrane surface at the same rate (since solids transfer rate per m2 membrane = flux x solids concentration).
It’s nonetheless surprising. Anyone operating a membrane thickener next to an MBR will doubtless have noticed that whereas the sludge in the MBR membrane tank looks pretty much like foaming bath water (only considerably dirtier), the sludge in the thickener looks more like boiling mud. It’s obviously thicker, but ‘gloopier’ as well: it seems to be almost a different material.
This is an example of the enigma that is sludge rheology. Rheology concerns the relationship between shear stress and shear rate. The shear stress is the force applied parallel to and at the liquid interface – as with, say, a skimmer. The shear rate γ is defined by how fast this force is applied. The ratio between shear stress and shear rate is the viscosity η.
For water, the viscosity doesn’t change with shear rate. For sludge, on the other hand, viscosity (or apparent viscosity ηa, to be specific) changes with pretty much everything: solids concentration, shear rate, temperature and, most likely, floc size and characteristics.
Many empirical models have been presented describing how the apparent viscosity of sludge changes with solids concentration. Most models (Table 1) predict an exponential increase in viscosity with MLSS concentration Xin accordance with the equation:
where the a−d are empirical constants which have been defined by various workers (Table 1). Equation can therefore be used to determine the apparent viscosity ηa for an applied shear rate γ for a given MLSS concentration X (Fig. 3), or as a function of MLSS concentration at different shear rates (Fig. 4).
Reference | a | b | c | d |
---|---|---|---|---|
Delgado et al, 2008 | 1.71 | 0.45 | −0.068 | 0.81 |
Laera et al, 2007 | 0.882 | 0.494 | −0.05 | 0.631 |
Pollice et al, 2007 | 1.94 | 0.262 | −0.124 | 0.359 |
Rosenberger et al, 2002 | 1.9 | 0.43 | −0.22 | 0.37 |
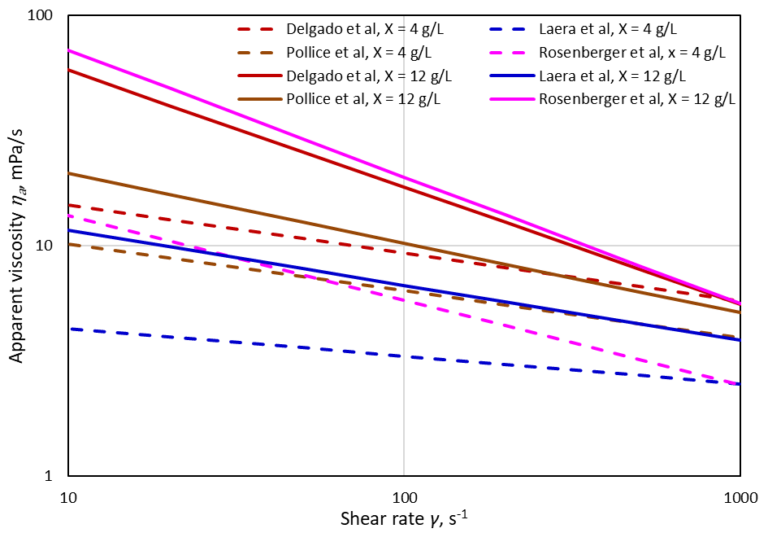
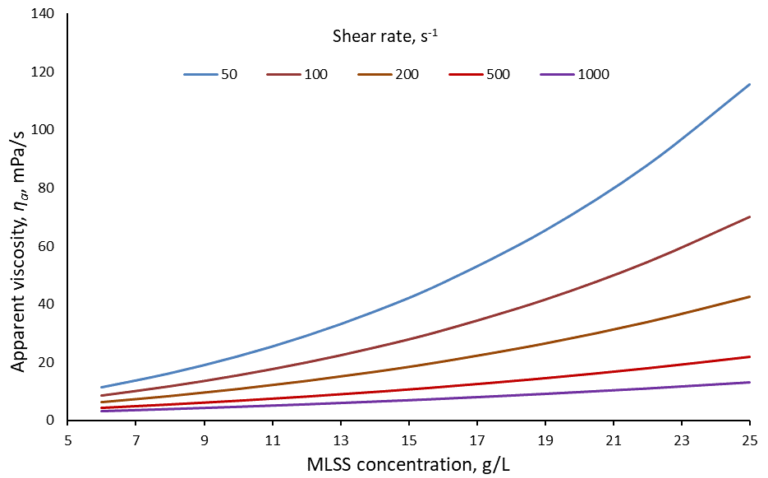
Regardless of the model, ηa increases with solids concentration and decreases with shear rate γ, but to varying extents depending on the assumptions made. In an iMBR with membrane air scour, γ is not well defined: it changes dynamically according to the way the air bubble passes across the membrane surface. So, it’s something of a challenge to decide exactly what value of γ to choose to determine ηa from the correlations given in Figs. 3−4.
What is clear, though, is that at the sort of solids concentrations used in membrane thickeners – well above 20 g/L – the apparent viscosities are significantly higher than for MBR sludges. This has been confirmed from many practical verification studies of viscosity, as summarised by Hong et al (2018).
And yet, despite these high sludge viscosities, it appears that the shear provided by the membrane air scour coupled with the more conservative imposed fluxes are sufficient to allow the process to be sustainable. This seems to be the case for solids concentrations as high as 40 g/L (i.e. 4 wt%), when the aerated sludge has taken on that ‘boiling mud’ appearance.
It is odd that there appear to be a paucity of scientific or technical publications which have unequivocally demonstrated sustainable operation of membrane sludge thickening of activated sludge, but this situation certainly isn’t unique. And if membrane thickeners aren’t limited by viscosity, provided the flux is kept sufficiently low, then one can only speculate as to the actual upper limit of the sludge concentration for this application and, by the same token, the MBR process.
That’s another research bid waiting to be written.