The integrated fixed-film activated sludge membrane bioreactor (IFAS-MBR): comparison with a regular MBR for nutrient removal
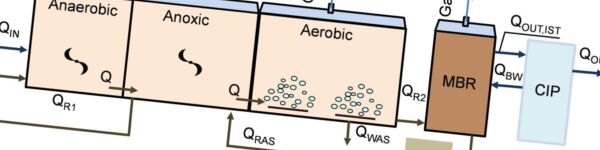
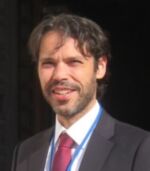
Giorgio Mannina (pictured)1,2*, George A. Ekama3, Marco Capodici1, Alida Cosenza1, Daniele Di Trapani1, Hallvard Ødegaard4
1Engineering Department, Università di Palermo, Viale delle Scienze, Ed. 8, 90100, Palermo, Italy
2Department of Earth and Environmental Engineering, Columbia University, 500 West 120th Street, New York, NY 10027, USA
3Water Research Group, Department of Civil Engineering, University of Cape Town, Rondebosch, 7700 Cape, South Africa
4NTNU − Norwegian University of Science and Technology, Department of Hydraulic and Environmental Engineering, 7491 Trondheim – Norway
* giorgio.mannina@unipa.it
1. Introduction
Biological nutrient removal (BNR) is achieved by combining anaerobic, anoxic and aerobic zones within a process, and transferring the mixed liquor between these zones to achieve the required biochemical reactions. The aerobic zone is normally based on the conventional activated sludge (CAS) process. The precise biological configuration with reference to the number and types of zones and the transfer between them varies between the different BNR configurations, and includes the UCT (University of Cape Town) process (Fig. 1).
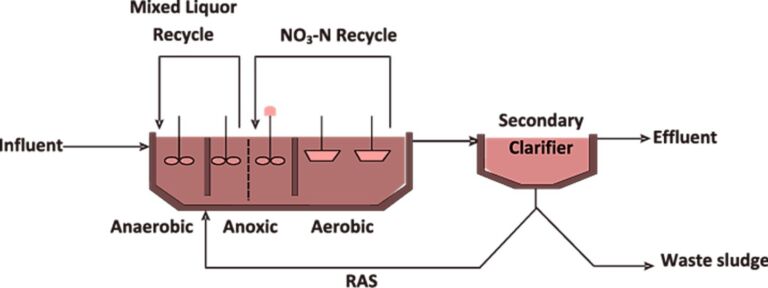
Nitrogen removal is usually achieved by the joint activity of ammonia oxidizing bacteria (AOB) and nitrite oxidizing bacteria (NOB) for nitrification, with the denitrification step conducted by heterotrophic organisms. Biological phosphorus removal (BPR) operates through phosphorus assimilation as intracellular polyphosphate by phosphorus accumulating organisms (PAOs) under alternating anaerobic/aerobic conditions.
The integrated fixed film activated sludge (IFAS) process represents an enhancement of CAS through the addition of media to the mixed liquor. This significantly reduces the required solids retention time (SRT) for nitrification, reducing the tankage accordingly, and also increases the amount of available carbon for denitrification through hydrolysis of the mixed liquor suspended solids (MLSS). This correspondingly increases the specific denitrification rate – by as much as a factor of two over CAS systems.
The use of media in MBR systems has been explored with reference to moving bed bioreactors (MBBRs). An MBBR-MBR system has been shown to increase the nutrient removal capability of the MBR alone due to the potential for simultaneous nitrification-denitrification within the biofilm fixed to the media. In addition, the use of media has been suggested to both reduce the sludge yield and membrane fouling compared with a conventional MBR.
The IFAS-MBR process is less well explored than the MBBR-MBR configuration. It is of interest to establish the performance of the IFAS-MBR when challenged with a variable influent C/N ratio, since this affects both nutrient removal and the emission of N2O – a highly active greenhouse gas (GHG). Its performance can then be compared with an MBR of the same process biological configuration for BNR, in this case the UCT process (Fig. 2).
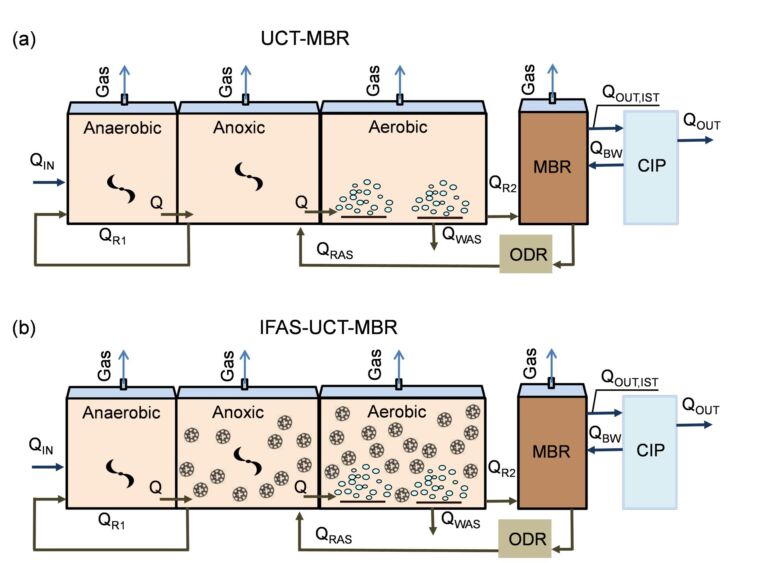
2. Materials and methods
The study was conducted at pilot scale on two UCT-configured BNR MBR plants (Fig. 1), one containing suspended Amitech plastic media (0.95 g cm-3 density, 500 m2 m-3 specific surface area) at a filling ratio of 40% and 15% in the aerobic and anoxic zones respectively. The anaerobic, anoxic, aerobic and membrane tanks were respectively 62, 102, 221 and 32 L in volume. The plants were each fed with municipal wastewater whose C/N ratio was adjusted by dosing with a sewage analogue (sodium acetate, glycerol, dipotassium hydrogen phosphate, and ammonium chloride).
The membrane used for each stream was a three-bundle Koch Puron hollow fibre module of 0.03 μm pore size and 1.4 m2 net area. The membrane was backwashed for 1 minute every 10 minutes. An oxygen depletion reactor (ODR) was used on the return activated sludge (RAS) stream to help maintain anoxic conditions in the anoxic tank.
The flows were as indicated in Table 1. Impacts of feedwater C/N ratios of (i) 5 and (ii) 10 mgCOD/mgN were studied, the solids retention time (SRT) values being 50 and 65 days at the lower ratio for the MBR and IFAS-MBR respectively and 40 days for both at the higher ratio.
Stream | Rate, L h-1 | |
---|---|---|
Influent and net permeate flow | QIN = QOUT | 20 |
Mixed liquor, anoxic to anaerobic tank | QR1 | 20 |
Mixed liquor, aerobic to MBR tank | QR2 | 100 |
MBR tank to anoxic tank, via oxygen depletion reactor (ODR) | QRAS | 80 |
The system was analysed for removal of COD, BOD, ammonia and nutrients, the latter being denoted by ηnit for nitrification, ηdenit for denitrification and ηNtotal for total nitrogen. A distinction was made between COD removal biologically and physically by the membrane.
Biokinetic parameters, specifically µH and µA for heterotrophic and autotrophic growth rates, were determined respirometrically. Samples were taken from the aerobic reactor.
Extracellular polymeric substances (EPS) were also measured, along with the soluble microbial product (SMP) content, and the protein and carbohydrate fractions of each determined. The sludge dewaterability was assessed with reference to the capillary suction time (CST) and specific resistance to filtration (SRF).
Enclosing the reactors allowed off-gas samples to be analysed for nitrous oxide (N2O).
Membrane fouling was monitored with reference to the ratio of the transmembrane pressure (TMP) to the flux and permeate viscosity (RT = TMP/(μP)). The total resistance RT was fractionated into the fouling resistance, comprising the pore plugging (RPB), and reversible and irreversible resistance contributions to cake layer fouling (RC,rev and RC,irr respectively).
3. Results and discussion
3.1 Removal data
Total COD removal efficiency was consistently above 98% for both configurations (Figs. 3a−b). Biological COD removal increased with increased C/N ratio (74 and 83% at C/N = 5 and 10 respectively for the MBR), and was higher for the IFAS-MBR at the lower C/N ratio value (80% vs 74% for the MBR).
For both systems nitrification efficiency increased with increasing C/N ratio (Figs. 3c−d). Both systems provided ~81% nitrification at C/N = 5, whilst at a C/N of 10 the MBR provided >95% nitrification compared with 91% for the IFAS-MBR. Since the IFAS-MBR was subject to a higher loading rate (805 cf. 674 mgNH4-N d-1), this configuration removed 14% more than the MBR in terms of ammonia load.
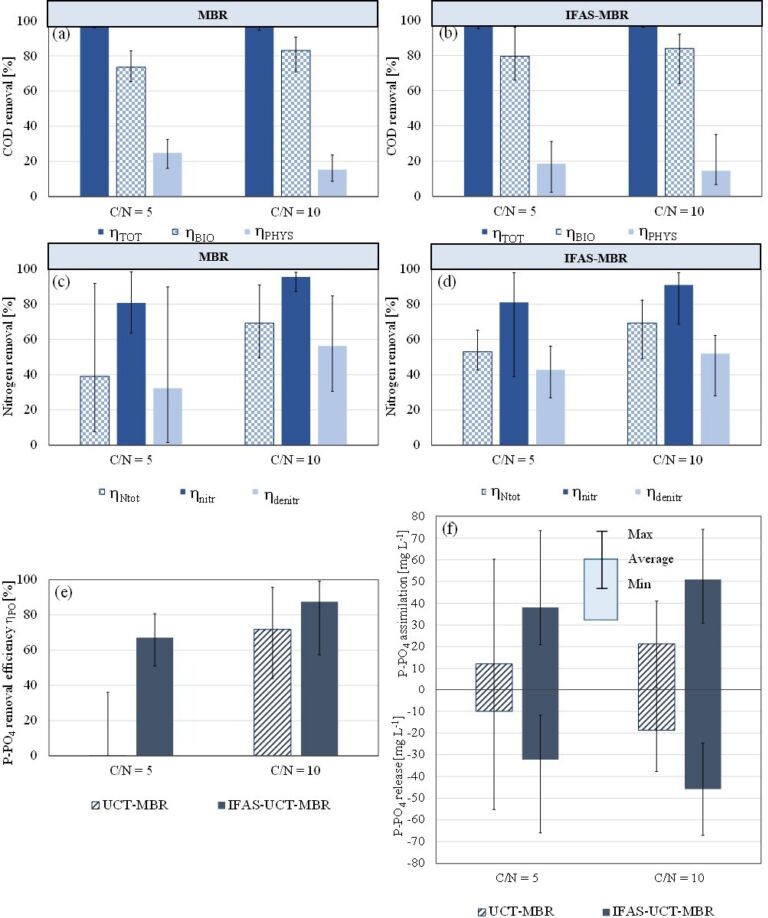
For the IFAS configuration, the nitrification rates at C/N = 5 were 4.3 and 4.8 mgNH4 L-1 h-1 for the biofilm and suspended biomass respectively, compared with 5.8 and 1.6 at the higher C/N ratio. This indicates the relative importance of the biofilm at higher C/N values and organic loadings: higher loadings promote competition between autotrophs and heterotrophs in the mixed liquor.
For denitrification and total nitrogen removal, performance increased with increasing C/N for both systems (Figs. 3c−d), with possible carbon limitation at the lower ratio. The IFAS-MBR nonetheless outperformed the MBR at this lower ratio (43% vs 32% denitrification, on average), indicating enhanced endogenous denitrification processes within the biofilm for the carrier-based system. As a result, total nitrogen (TN) removal was consistently higher for the IFAS-MBR, especially at the lower C/N ratio (53% vs 39%).
Data for mean P-PO4 removal (Fig. 3e) and mean P concentration released/assimilated (Fig. 3f) for each configuration indicated severe carbon limitation at C/N = 5 for the MBR, impeding biological denitrification such that the nitrate concentration recycled to the anaerobic reactor was significant. Under these conditions, the anaerobic reactor behaves as an anoxic reactor, subsequently negating biological P removal. The IFAS-MBR was much less subject to this limitation providing 65% P removal at C/N = 5 compared with zero for the MBR.
3.2 Respirometric data
For heterotrophic species (mixed ordinary heterotrophs and PAO) in the suspended biomass, the IFAS media generated enhanced activity (reflected in higher μH and SOUR values, Figs. 4a−b) compared with the pure MBR system. This suggests specialisation/adaptation of the IFAS biomass, reducing competition between the suspended biomass and the biofilm for the availability of the different substrates. In contrast, heterotrophic activity within the biofilm (the light blue blocks in Figs. 4a−b) was much lower. As expected, heterotrophic activity was considerably higher than autotrophic activity (μ = 2.2−8.2 d-1, Fig. 4a, cf. 0.25−0.95 d-1, Fig. 4c).
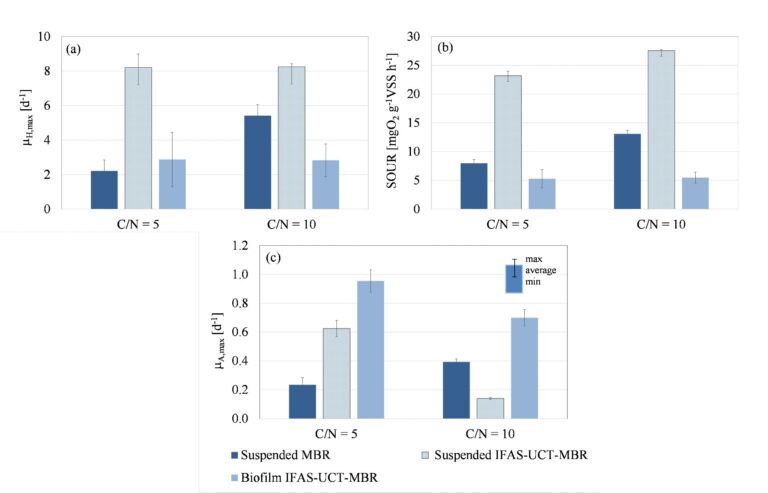
Fig. 4c reveals the differing autotrophic activity (mixed AOB and NOB), between the two systems. Much higher nitrifier growth rates (μA,max) arise at C/N = 5 for the IFAS-MBR compared with the MBR alone, particularly in the biofilm (0.96 cf. 0.24 d-1). This is attributable to the longer SRT of the IFAS-MBR, which also impacts on the suspended biomass μA,max which, at 0.64 d-1, is more than 2.5 times higher than the corresponding MBR value.
At C/N=10 the autotrophic activity for the IFAS-MBR suspended biomass decreased significantly, and was lower than that for the pure MBR. This may reflect the impact of heterotrophic organisms occluding the nitrifiers on the carrier due to the greater availability of biodegradable organic matter. However, within the biofilm itself the denitrification is still faster for the IFAS-based system than for the MBR flocs.
3.3 Sludge dewaterability
CST and SRF data (Table 2) indicate that the IFAS-MBR sludge is less readily dewaterable than the MBR sludge, reflecting the impact of the detached biofilm. The overall mean CST for the MBR sludge was 17 s (ranging from 15 to 20 s), compared with 25 s (14−41s) for the IFAS-MBR, and the corresponding mean SRF values 3.3 x 1012 (2.7−4.1 x 1012) and 4.6 x 1012 (3.5−5.9 x 1012) m.kg-1.
Parameter | Reactor |
MBR: C/N = 5 |
MBR: C/N = 10 |
IFAS-MBR: C/N = 5 |
IFAS-MBR: C/N = 10 |
---|---|---|---|---|---|
CST, s | Anaerobic | 14.9 (1.2) | 15.1 (1.0) | 16.0 (2.2) | 14.0 (4.2) |
Anoxic | 17.7 (1.8) | 18.6 (1.7) | 25.0 (7.5) | 21.5 (8.5) | |
Aerobic | 16.9 (4.3) | 16.1 (1.4) | 22.6 (6.3) | 23.2 (6.0) | |
MBR | 18.0 (2.0) | 19.9 (1.8) | 41.3 (14.4) | 34.2 (13.8) | |
- | - | - | - | - | - |
SRF, 1012 m∙kg-1 | Anaerobic | 2.7 (0.8) | 3.3 (0.8) | 5.3 (4.5) | 5.3 (1.4) |
Anoxic | 2.8 (0.8) | 4.1 (0.6) | 4.1 (0.7) | 5.9 (2.4) | |
Aerobic | 3.0 (0.4) | 4.0 (0.9) | 4.0 (1.7) | 3.5 (1.7) | |
MBR | 2.8 (0.8) | 3.6 (0.6) | 4.8 (1.4) | 3.6 (3.1) |
3.4 Extracellular polymeric substances
Fractionation of the biomass EPS (bound organics) and SMP (unbound), expressed as a percentage of the total, revealed the protein fraction of the EPS (EPSP) to be the most significant across both conditions and for both plant configurations (Fig. 5). The SMP fraction was insignificant for the MBR but made up between 13 and 21% of the total for the IFAS-MBR with the protein fraction (SMPp) predominating.
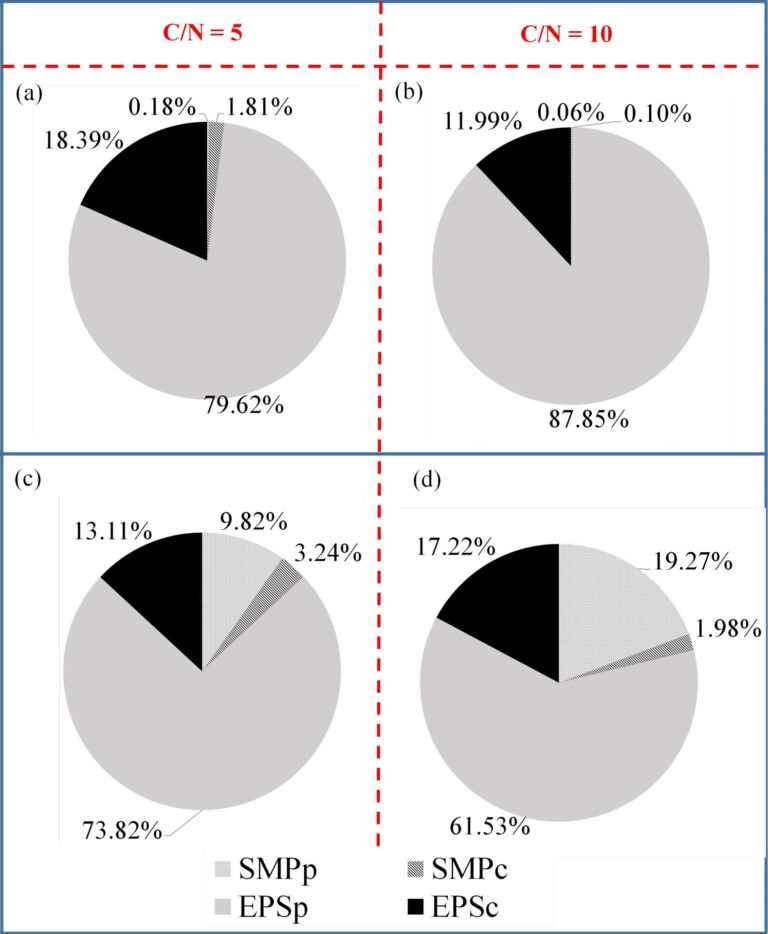
3.5 Membrane fouling
Fouling transients for both configurations (Figs. 6a−b), depicted as total membrane resistance (RT) and fouling rate (FR), indicate reduced fouling for the MBR compared with the IFAS-MBR. This is manifested as reduced cleaning frequency (the vertical dashed red lines in Figs. 6a−b), and a lower mean RT value (the horizontal dot−dashed lines), determined as 5.4 x 1012 cf. 9.0 x 1012 m-1 for the MBR and IFAS-MBR respectively at the highest C/N ratio.
Foulant fractions were determined for steady-state operating conditions for both configurations. At a C/N ratio of 10 around 98% of the fouling could be attributed to irreversible cake deposition (RC,irr) for both processes. This was also true of the MBR at the lower C/N ratio. For the IFAS-MBR at the lower C/N ratio, however, around 18% of the fouling could be attributed to pore blocking, which may have contributed to the increased fouling propensity of this configuration. This behaviour would appear to contradict the supposition that the scouring action of the media may suppress fouling in the IFAS-MBR.
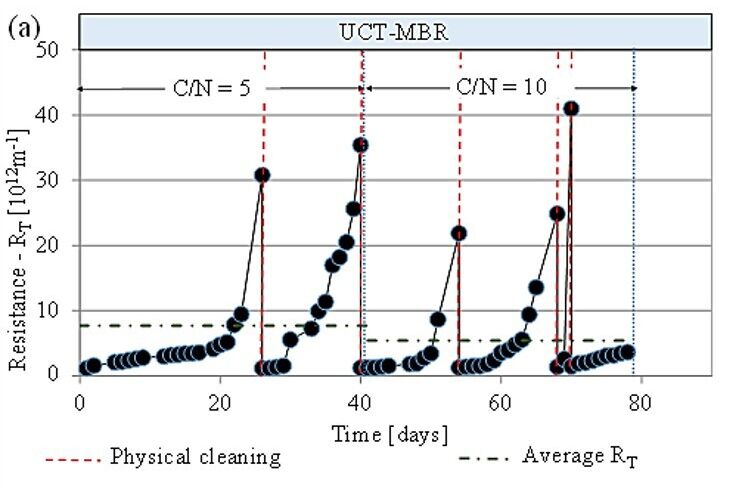
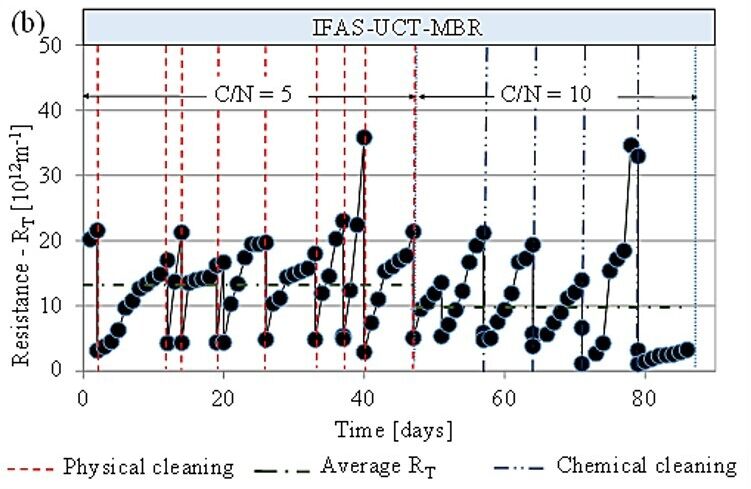
3.6 N2O emissions
Average gaseous nitrous oxide concentrations (Fig. 7) indicated a highest average concentration of 71 µg N2O-N L-1 for the aerobic reactor of the IFAS-MBR at the higher C/N ratio. This compared to a maximum value of 54 µg N2O-N L-1 for the anaerobic tank of the MBR at the lowest C/N ratio. Gas concentrations were somewhat lower than the measured liquid-phase concentrations, for which the maximum values were between 100 and 340 µg N2O-N L-1. The increased N2O production at low C/N ratios is a consequence of the limitation in the nitrogen transformation process associated with low carbon availability.
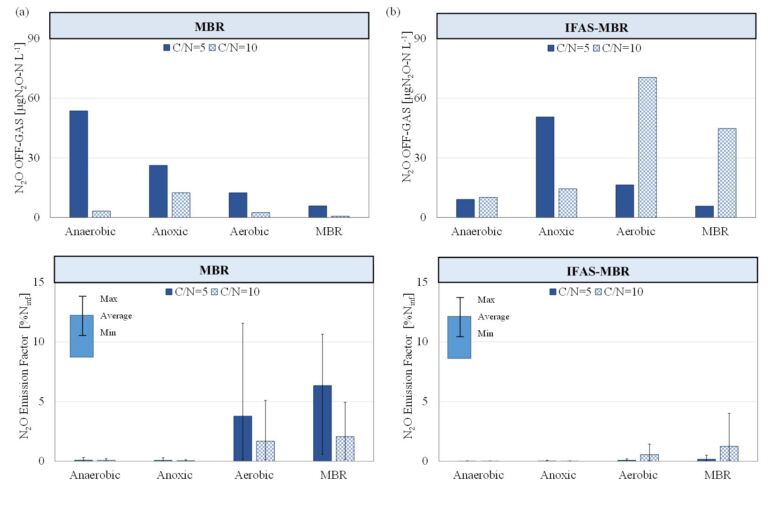
The higher N2O concentrations measured for the IFAS-MBR do not translate to higher emission factors. The emission factors, i.e. the % of influent N emitted as N2O, are significantly affected by the aeration devices which strip the dissolved N2O from the sludge. Thus a significantly higher proportion of the influent N (3.5% cf. 0.5%) arises as N2O in the offgas for the MBR compared to the IFAS-MBR.
4. Conclusion
An experimental campaign aimed at comparing an MBR and IFAS-MBR configured for biological nutrient removal at two different influent C/N ratios has revealed:
- COD removal was comparable for the two configurations, removal being consistently above 98% for both configurations
- total nitrogen (TN) removal was consistently higher for the IFAS-MBR, especially at the lower C/N ratio of 5 where a mean value of 53% was recorded compared with 39% for the MBR
- at the lower C/N ratio phosphorus removal was less vulnerable to carbon limitation for the IFAS-MBR than for the MBR
- the nitrous oxide emission factor was lower for the IFAS-MBR, particularly at low C/N ratios, reflecting the role played by biofilm in mitigating the N2O emission
- membrane fouling was more pronounced for the IFAS-MBR.
Compared with a conventional MBR the IFAS-MBR configuration may offer a more robust treatment solution with reduced greenhouse gas emissions at low C/N ratios.