Sludge viscosity − the thick of it
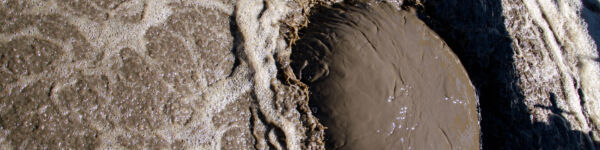
Tamas Zsirai1, Pompilia Buzatu1, Roberta Maffettone2 and Simon Judd1
1 Cranfield University
2 University of Salerno
1. Introduction
The focus of a significant section of the MBR scientific community from the late 90s onwards has centred on the idea of some measurable MBR sludge quality which can be used to predict permeability, preferably through on-line measurement. Hundreds of papers have been produced based on correlation of membrane fouling with specific candidate foulant species − chemicals, biochemicals or particles of a specific size range. In general, whilst some correlations have been produced successfully, none extend across multiple full-scale plants. There are a number of possible explanations for this, but perhaps the most obvious of these is that bulk sludge filterability depends on more than just chemistry and biochemistry, and specifically on the multifarious and very complex impacts of the microbial ecology.
On occasions, researchers have taken time off from characterising foulant chemistry in MBR sludge to take a look at its bulk properties. There are a number of excellent publications on the subject from various research groups around the world, including studies of pilot and full-scale plants. Such studies can be roughly divided into those generating correlations between:
- flux, permeability and fouling rate with a sludge bulk property (viscosity or temperature), or
- a sludge bulk property (viscosity) with another sludge bulk property (temperature, concentration or composition).
In practice, a rough-and-ready indication of bulk sludge quality is provided by (specific) sludge volume index ((S)SVI), capillary suction time (CST) and time to filter (TTF). While it may be presumed that the filtration-based parameters of CST and TTF may provide a reasonable basis for determining the membrane permeability, it is likely that the simple SSVI measurement is a better measure. High-SSVI sludges are often associated with filamentous micro organisms, which are widely known to generate a high resistance to sludge filtration due in part to the hydrophobic nature of the micro organisms.
So, just how do sludge filterability and viscosity change with the key property of temperature? This is possibly the simplest, and the most ubiquitous, relationship to consider. The rheological properties of both water and sludge are widely understood. Whilst water behaves as a simple Newtonian fluid, with a temperature dependence which is absolutely predictable, sewage sludge displays thixotropic properties (Seyssiecq et al, 2003) and is often defined rheologically as a Bingham plastic whose apparent viscosity changes with both shear rate and solids concentration (Yang et al, 2009).
2. MBR sludge viscosity
The non-Newtonian nature of sewage sludge means that its measured viscosity µs, referred to as 'apparent viscosity', varies with shear rate due to the shear-dependent deformation of the flocculant solids (Yang et al, 2009). It stands to reason that the viscosity affects the shear at the membrane interface, shear being a key parameter in promoting flux, and that the dependency of apparent viscosity on temperature may then also be significant in determining flux.
The change in the viscosity of water (in mPa s) with temperature within the limits of 5 and 35°C can, within an R2 value of 0.9995, be represented by the quadratic equation:
Several authors have offered empirically-derived correlations between viscosity and temperature for sludge. Correlations have been offered which incorporate solids concentration, including that provided by Psoch and Schiewer, 2008:
as well as the expression of Yang et al, (2009):
where Rg is the universal gas constant (8.316 J/(mol.kg)) and E the activation energy for viscosity, 9.217 kJ/mol.
Alternative forms proposed for constant MLSS conditions include the de Andreade form of the viscosity:temperature relationship (Rodríguez et al, 2010):
where proposed values include A = 7.17 and B = 1.09, but for which the authors own data suggest values of 2.31 and B = 11.21 respectively. There is also the equation suggested by Roorda and van der Graaf (2000):
where T is the temperature in °C.
In fact, two of the trends (Equations 2 and 5) provide the same trend as that of water (Fig. 1); whilst the absolute viscosity value is obviously dependent on the MLSS concentration, the impact of temperature is apparently viewed as being the same as for pure water. This is perhaps not unreasonable, given that it is only water that actually flows through the membrane. However, the resistance to this flow of permeate is primarily attributed to the gelatinous cake layer of material at the surface of the membrane. Assessing the change in the nature of this layer with temperature is perhaps too difficult. However, it is also the case that the sludge rheology probably has little impact on the flow through the membrane in any case.
The impacts of sludge concentration, on the other hand, are more evident. Equations 2 and 3 respectively predict a linear and exponential increase with MLSS concentration. Various studies have shown such viscosity increases through practical measurement, most recently Rodríguez et al, (2010) and, in an exhaustive study encompassing many full-scale installations, Moreau et al, (2009). Interestingly, the latter authors found no discernible impact of temperature across the many installations examined due to widespread data scatter (Fig. 2b), perhaps calling into question the validity of applying any kind of temperature-related viscosity correction.
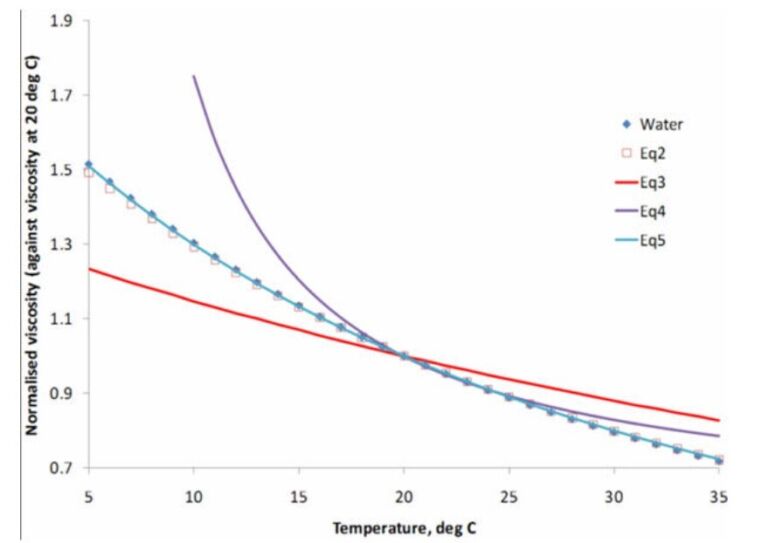
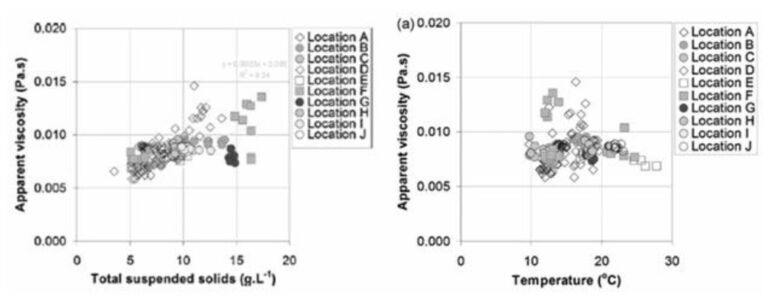
3. Temperature impacts on permeability
The key impacted parameter from temperature changes is, of course, the flux. Data from pilot or full-scale installations which might verify any trend between permeability and temperature appear to be rare. However, there are two studies which provide a valuable insight into temperature effects: van den Brink et al (2011) and Moreau et al (2009).
The first of these report data from a single plant (Varsseveld), whilst the second concerned a number of different installations. van den Brink and her co-authors were able to identify a clear pattern in permeability decreasing with both flux and temperature over and above what would be expected from the standard viscosity correction of Equations 1, 2 and 5 (Fig. 3a), similar in nature to the change in viscosity proposed by Rodriguez et al, 2010 (Fig. 3b). This being the case, then it would seem that for a single installation it is appropriate to apply a correction for temperature which exceeds that relating just to water viscosity changes (the blue line in Figure 1), since the plots given in Figure 3a are temperature-corrected for this.
However, the extensive study by Moreau et al, across a number of European plants would seem to indicate that temperature impacts generally are barely significant, and that wide ranging filterability values are evident despite a very narrow range of viscosity (Fig. 4). Thus, whilst there may be some temperature-related sludge property which impacts on filterability, this is (a) not limited to bulk viscosity, and (b) largely eclipsed by the impact of other sludge quality parameters.
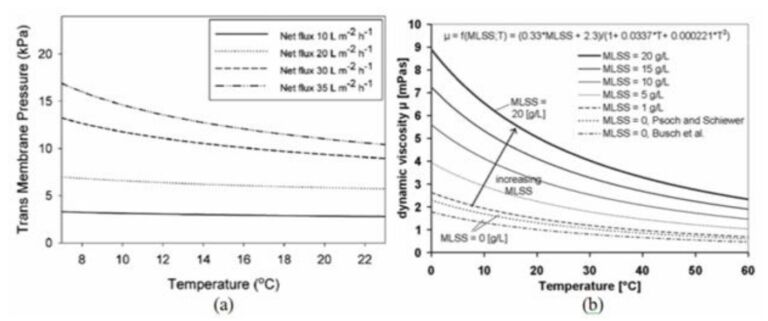
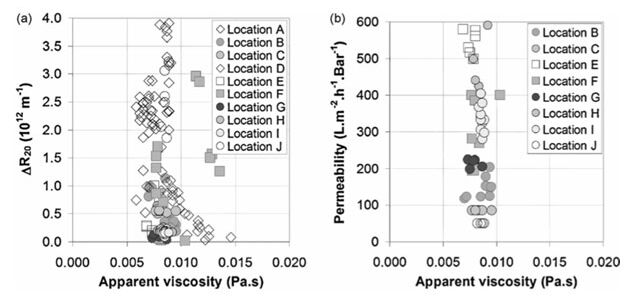
4. And finally…
So, if bulk properties of the sludge have minimal impact on permeability, and the fractionation of sludge to discern the chemistry of individual foulants is becoming increasingly questionable (as the theses of Adrien Moreau and his successor at TU Delft, Maria Lousada-Ferreira, would seem to suggest), then what is it that causes such huge changes in sludge filterability as indicated in Figure 4? And why doesn’t sludge filterability correlate with the actual MBR permeability?
Clearly, as far as the latter is concerned, the condition of the membrane (how permanently fouled or clogged) will impact on the apparent permeability simply due to occluded membrane area. However, the wide variations in bulk sludge filterability are less easily explained. This is most evident from the poor correlations reported, by both Moreau and Lousada-Ferreira, between the measured sludge filterability and concentrations of individual candidate foulants such EPS, SMP, colloid concentration.
There is, it seems, much to learn about MBR sludge quality and its impact on sustainable MBR operation. Despite the equivocal nature of the evidence for improved permeabilities at higher temperatures, anecdotal evidence from full scale plants suggests that plants operating in warm climates tend to yield higher fluxes than those out in the cold. It would also be expected for the chemical clean to be more effective at higher temperatures. Regardless of the precise benefit of elevated temperatures and good physical sludge quality, there remains an imperative of maintaining favourable biological conditions.
Perhaps the best we can provide at the moment in terms of sludge quality monitoring is assessment of (i) settleability by SSVI (ii) maintenance of nitrification, and (iii) levels of the hateful filamentous bacteria. Whilst this won’t provide an absolute guarantee of trouble-free operation, it’s perhaps as good as we can do at the moment − that and staying warm.